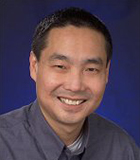
PL-1 Extracellular Electron Transfer Mechanisms between Microorganisms and Iron-bearing Minerals and Their Biotechnological Applications
Liang Shi
Department of Biological Sciences and Technology, School of Environmental Studies, China University of Geosciences, Peoples Republic of China
Iron (Fe) is the fourth most abundant element in the Earth’s crust where ferrous Fe [Fe(II)] and ferric Fe [Fe(III)] can be used by microorganisms for energy conservation. The Fe(III)-reducing microorganisms couple oxidation of organic matter, H2 and methane to Fe(III) reduction for anaerobic respiration, while the Fe(II)-oxidizing microorganisms couple Fe(II) oxidation to reduction of O2, NO3 and CO2 for growth. Microbial redox cycling of Fe(II/III), thus, plays important roles in global cycling of not only Fe but also carbon and nitrogen. At the circumneutral pH and under oxic condition, Fe(II) is quickly oxidized to Fe(III) abiotically that is readily precipitated to solid Fe(III) (oxyhydr)oxides in the absence of any chelator. Thus, Fe-bearing minerals are abundant in environment. The transfer of electrons between redox carriers in the microbial cytoplasmic membrane and Fe-bearing minerals external to the cells is often referred to as microbial extracellular electron transfer.
As a key component of microbial cell envelope, the cytoplasmic membrane is the primary barrier to the external environment and the center of electron transfer reactions, which is essential for microbial energy generation. The microbial cell envelope, however, often includes other structural components external to the cytoplasmic membrane, such as the peptidoglycan, outer membrane and S-layer, which are electrically nonconductive and physically impermeable to Fe-bearing minerals. To overcome the electrical and physical barrier external to the cytoplasmic membrane, microorganisms have evolved distinct mechanisms for electron exchange between the cytoplasmic membrane and extracellular Fe-bearing minerals via a network of redox and structural proteins. These proteins often form pathways that electrically and physically connect the intracellular metabolic processes with the redox transformations of extracellular mineral-associated Fe ions. Over the past 18 years, substantial progress has been made in identification and characterization of microbial extracellular electron transfer pathways. Among them, the metal-reducing pathway of Shewanella oneidensis MR-1 is the most rigorously investigated and best characterized microbial extracellular electron transfer pathway. The microorganism with extracellular electron transfer capabilities have also been employed for a variety of biotechnological applications, ranging from contaminant remediation in environment to production of energy and the nanomaterials with novel properties.
As a key component of microbial cell envelope, the cytoplasmic membrane is the primary barrier to the external environment and the center of electron transfer reactions, which is essential for microbial energy generation. The microbial cell envelope, however, often includes other structural components external to the cytoplasmic membrane, such as the peptidoglycan, outer membrane and S-layer, which are electrically nonconductive and physically impermeable to Fe-bearing minerals. To overcome the electrical and physical barrier external to the cytoplasmic membrane, microorganisms have evolved distinct mechanisms for electron exchange between the cytoplasmic membrane and extracellular Fe-bearing minerals via a network of redox and structural proteins. These proteins often form pathways that electrically and physically connect the intracellular metabolic processes with the redox transformations of extracellular mineral-associated Fe ions. Over the past 18 years, substantial progress has been made in identification and characterization of microbial extracellular electron transfer pathways. Among them, the metal-reducing pathway of Shewanella oneidensis MR-1 is the most rigorously investigated and best characterized microbial extracellular electron transfer pathway. The microorganism with extracellular electron transfer capabilities have also been employed for a variety of biotechnological applications, ranging from contaminant remediation in environment to production of energy and the nanomaterials with novel properties.
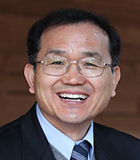
PL-2 Redox Sensors Enhancing Thermo-Tolerance in Plants
Sang Yeol Lee
Department of Life Science, Gyeongsang National University
A variety of redox proteins are distributed in diverse subcellular organelles in plants including the chloroplast, cytoplasm, mitochondria and nucleus, despite their physiological functions are largely remained to be cleared. For the protection of plants from external stresses that produce various kinds of reactive oxygen species (ROS), Redox Sensors regulate diverse intracellular signaling cascades and play critical functions. Considering the important criteria required to be Redox Sensors, in our lab, more than 30 Redox-Sensor candidate proteins possibly playing highly important roles have been identified from Arabidopsis. Thus, in this seminar, we will introduce the molecular and functional characteristics of the three representative Redox-Sensors and their physiological roles in plant defense systems. At first, we will present on the novel function of H2O2-catalyzing peroxidase, 2-Cys peroxiredoxin, which can have additional function of molecular chaperone under the oxidative and heat shock condition. To carry out the dual functions acting both as a peroxidase and as a molecular chaperone, the protein should reversibly change its protein structures from oligomer to dimer. In addition, because the protein chaperone generally works as a regulator of circadian clocks, next, we will present the clock-regulating function of the chaperone. Finally, we select a typical enzyme of cytosolic type of redox protein, Red-P, and studied in associated with cold-stress resistance in plant. To elucidate its physiological functions in plants, we screened its interaction partners by yeast 2-hydrid assay and co-immunoprecipitation experiments. From the experiment, we found a quite interesting result that the cytosolic Red-P interacted with nuclear CBFs, cold-responsive transcription factors, that play critically important functions in cold resistance in plants. The results prompted us to investigate its subcellular localization and functional roles in respect to freezing stress in plants. Our study demonstrated that cold-mediated nuclear translocation of cytosolic Red-P strongly binds with CBFs and regulate their structural conformation with a redox-dependent manner, which activates their transcriptional activity to express downstream cold-responsive COR genes. Therefore, overexpression of Red-P greatly enhances freezing tolerance in plants. In conclusion, it is possible to conclude that the multiple stress-tolerant useful crops can be prepared by the regulation of the gene expression encoding Redox Sensors in plants.